Seawater desalination by solar evaporation
Electrostatic repulsion for electricity generation
Solar desalination presents an exciting opportunity to simultaneously produce fresh water and generate electricity using sustainable methods. During my PhD research, I explored the use of alginate-based composites, crosslinked with multivalent cations like aluminum and zirconium, to recover lithium from seawater. These materials exhibited a surprising ability to repel dissolved cations—an effect that led me to ask a new question: Could the repulsive ionic forces within the alginate matrix create an internal salinity gradient strong enough to generate electricity during solar evaporation? This post introduces the scientific background behind that idea, which merges seawater desalination, ion-selective membranes, and solar energy into a unified system. Further details on lithium recovery applications will follow in a future post.
Electricity is generated through the creation of an electrical potential difference. In the context of solar desalination and evaporation-driven systems, electricity can be harvested when electrolytes, such as seawater, move through a nanoporous membrane or charged microchannel. For efficient energy conversion, the size of these channels must be close to the Debye length of the fluid—allowing the formation of an electrical double layer (EDL) by counterions responding to the negatively charged surface.
As evaporation-induced hydrodynamic pressure drives fluid through the nanopores, the mobile counterions are carried downstream. This movement of charge generates a potential difference known as the streaming potential, which can be harnessed for power generation. For a more in-depth explanation of this mechanism, refer to the work of Dr. Jun Hong Park, who conducted a parallel study on evaporation-induced energy harvesting from seawater.
In contrast, alginate composites crosslinked with multivalent cations inherently exhibit ion-repulsive properties toward seawater cations. During the evaporation process, water molecules migrate to the upper surface and undergo phase transition, while the transport of cations is suppressed due to electrostatic interactions with the crosslinked matrix. This mechanism results in the establishment of a vertical salinity gradient, characterized by a lower ionic concentration near the evaporation surface compared to the base. The resultant ionic disequilibrium gives rise to an electrical potential difference. The magnitude and configuration of this gradient are influenced by the specific type of crosslinking cation employed, as illustrated in the schematic below.
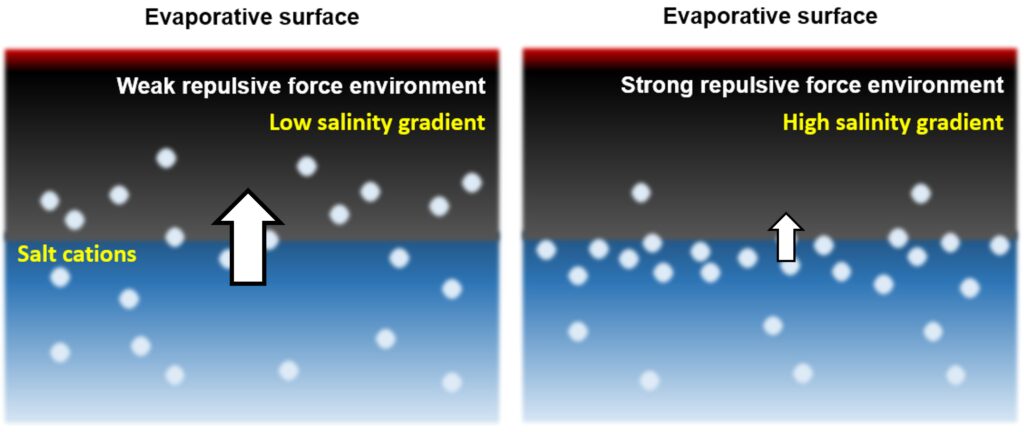